Skip to:
- Reading List and Case Studies
- Regulatory and Policy Examples
Introduction
Energy storage systems are technologies capable of charging energy from an external source and discharging this energy at a later time. The emergence of storage technologies, such as grid-scale battery energy storage systems (BESS), has created new opportunities for shifting energy supply and demand. This unique ability of energy storage can facilitate the integration of renewable energy resources through the provision of several types of services.
Location
Energy storage systems can be broadly categorized based on 1) where they are interconnected (e.g., in front-of-the-meter, behind-the-meter, or off-grid) and 2) the type of energy they store (e.g., thermal, mechanical, electrochemical, etc.). Where storage systems are interconnected has important implications for who owns them, what technology they use, their size and what services they are capable of providing:
- Behind-the-meter (BTM) - these are small systems usually located directly at the customer premises, which directly interconnect to the distribution system. Often these systems are used to provide backup power or power-quality-related services to the system owner. However, as communication, telemetry and inverter equipment improve, these systems are increasingly being used to provide distribution level services. In some advanced markets, distribution systems are also being aggregated to provide transmission-level, wholesale market services.
- Grid-scale, distribution systems – these systems are much larger than BTM systems and supply services to the distribution or transmission system, such as regulation reserves, load following or upgrade deferrals.
- Grid-scale, transmission systems– these systems tend to be the largest and are connected at the transmission level to provide services such as regulation or load following.
- Off-grid systems – these systems are isolated from and operate independently of the grid.
Technology Type
The technology used to store energy also has important implications for the types of services systems can supply. Each technology, whether mechanical systems like pumped hydroelectric storage or electrochemical systems like lithium-ion batteries, will have set characteristics and unique advantages and disadvantages that make them suitable for different grid system applications. A table of definitions for a few of these characteristics are shown below.
Table 1: Definitions of Key Battery Technical Characteristics
Technical Characteristic |
Definition |
Rated Power Capacity |
Total possible instantaneous discharge capability (in kilowatts [kW] or megawatts [MW]) of the BESS, or the maximum rate of discharge that the BESS can achieve, starting from a fully charged state |
Energy Capacity |
Maximum amount of stored energy (in kilowatt-hours [kWh] or megawatt-hours [MWh]) a battery can hold |
Energy/Power Density |
Measure of the energy or power capacity of a battery relative to its volume (kW/L, kWh/L) |
Specific Energy/Power |
Measure of the energy or power capacity of a battery relative to its weight (kW/g, kWh/g) |
Storage Duration |
Amount of time storage can discharge at its power capacity before depleting its energy capacity |
Cycle Life/Lifetime |
Amount of time or cycles a battery storage system can provide regular charging and discharging before failure or significant degradation |
Round-trip Efficiency |
Ratio of the energy charged to the battery to the energy discharged from the battery |
Self-discharge |
Reduction of stored energy of the battery (% of charge/time) through internal chemical reactions, rather than through discharging to perform work. |
A brief explanation of the various technologies is given below. Readers interested in a more detailed overview of these technology types can explore the DOE’s Electricity Storage Handbook or the Asian Development Bank’s Handbook on Battery Energy Storage System.
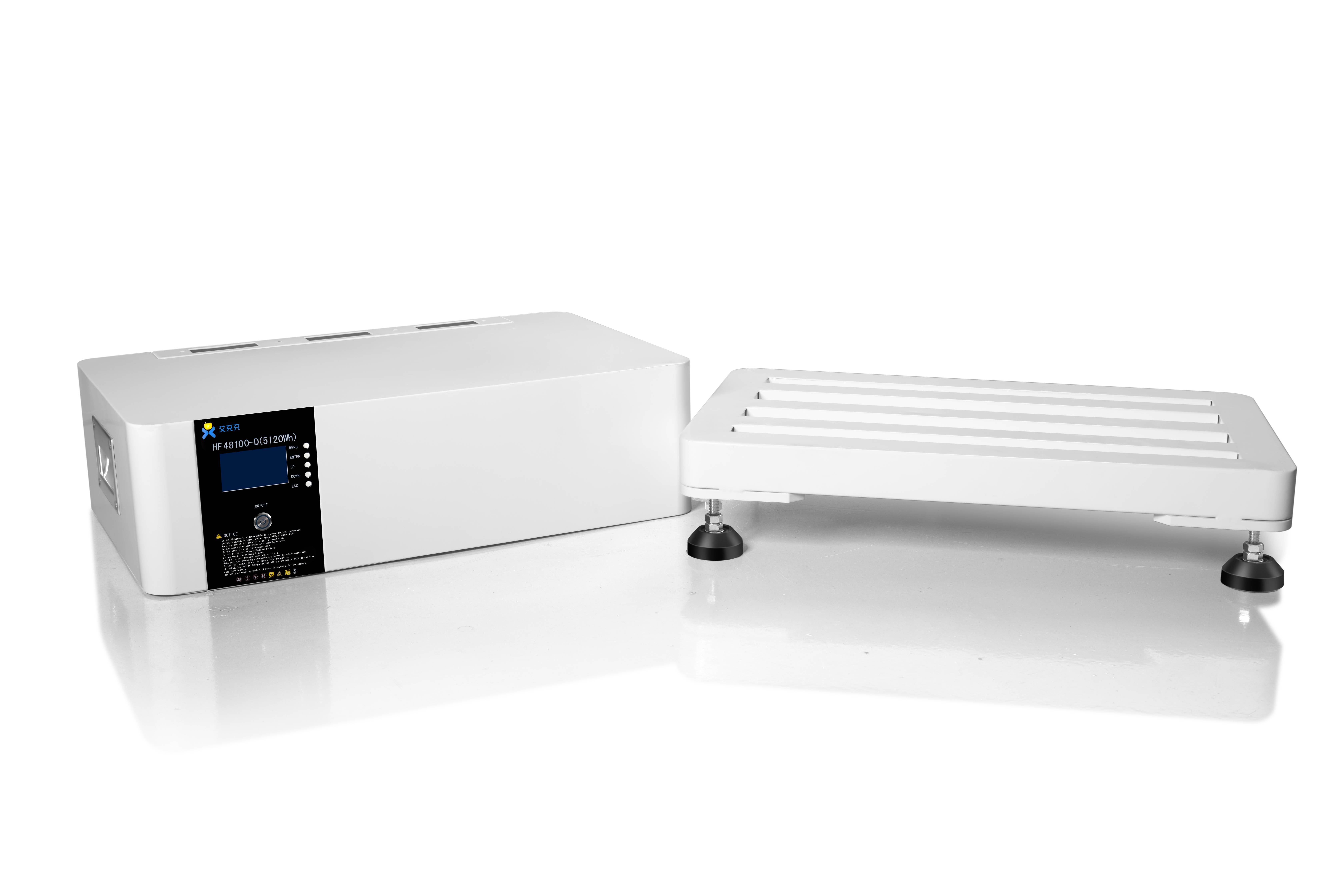
- Thermal energy storage systems – these operate by creating a temperature gradient or by inducing a material phase change anywhere from hours to seasons. They are primarily used in large, front-of-the-meter installations. When used for storing and generating electricity, as opposed to meeting heating and cooling demands, are typically paired with concentrated solar power (CSP) plants and use molten salt as a medium.
- Mechanical energy storage systems – these systems transform potential and/or kinetic energy into electricity. Examples include pumped hydroelectric energy storage (PHES), compressed air energy storage (CAES) and flywheels. PHES and CAES are typically used in very large, front-of-the-meter transmission-level installations and are mainly used for load-following applications. Flywheels, however, may also be used in behind-the-meter applications such as uninterrupted power supply.
- Electrical energy storage systems – these are short-duration systems that store electricity in the electric field of supercapacitors or in the magnetic field of superconductors. In the power sector, these are primarily used to maintain a high level of power quality.
- Electrochemical storage systems – these generate electricity through reversible chemical reactions. Battery energy storage systems (BESS) are the most common and commercial form of this technology and are widely deployed at the behind-the-meter, distribution and transmission levels. Battery technologies are classified based on different chemistries and technical properties and include lithium-ion (currently the market leader for a wide variety of applications); lead-acid; nickel-based; sodium-based; and flow batteries. Each of these chemistries varies strongly with respect to technical characteristics and costs and are accordingly used in a wide variety of applications.
Storage Services
Energy storage systems are capable of providing a wide range of system services depending on where they are interconnected and their technical characteristics (which are, in part, determined by their technology type). These services can be broadly categorized as:
- Providing capacity services and energy shifting: System operators must ensure they have adequate supply of generation capacity to reliably meet demand during the highest-demand periods in a given year. This peak demand is typically met with higher-cost generators, such as gas plants; however, depending on the shape of the load curve, energy storage systems can also be used to ensure adequate peaking generation capacity. System operators can also improve the ability of variable renewable energy (VRE) plants to contribute to peaking capacity by pairing VRE with energy storage. Pairing VRE resources with energy storage can enable these resources to shift their generation to times when it is needed most.
- Providing fast-response ancillary services: Many forms of energy storage, notably batteries, are capable of rapidly and accurately changing their charging and discharging rates in response to external signals. By quickly changing their output, these storage resources can provide valuable ancillary services that system operators use to help balance differences between demand and supply. These ancillary services are particularly important in systems with large amounts of variable renewable energy generation, as system operators must be able to respond to unexpected changes in energy supply. Currently, ancillary services are predominantly provided by partially loaded conventional generators. Using energy storage to align supply and demand as well as to provide ancillary services can increase the flexibility of the grid and help reduce the curtailment of renewable energy resources and spinning reserve requirements from conventional resources.
- Transmission and Distribution Upgrade Deferral: The electricity grid’s transmission and distribution infrastructure must be sized to meet peak demand, which may only occur over a few hours of the year. When anticipated growth in peak electricity demand exceeds the grid’s existing capacity, costly investments are needed to upgrade equipment and develop new infrastructure. Deploying energy storage can help defer or avoid the need for new grid investments by meeting peak demand with energy stored from lower-demand periods, thereby reducing grid congestion and improving overall transmission and distribution asset utilization.
- Black Start: When starting up, large generators need an external source of electricity to perform key functions before they can begin generating electricity for the grid. During normal system conditions, this external electricity can be provided by the grid. After a system failure, however, the grid can no longer provide this power, and generators must be started through an on-site source of electricity. On-site energy storage such as a lithium-ion battery storage system can provide this service, avoiding fuel costs and emissions from conventional black-start generators. As system-wide outages are rare, on-site energy storage can provide additional services when not performing black starts.
Some energy storage systems, in particular Battery Energy Storage Systems (BESS), can maximize their value to the grid and project developers by providing multiple system services. As some services are rarely called for or used infrequently in a given hour, designing BESS to provide multiple services enables a higher overall battery utilization. This multi-use approach to energy is known as value-stacking. For example, a BESS project can help defer the need for new transmission by meeting a portion of the peak demand with stored energy during a select few hours in the year. When not meeting peak demand, the BESS can earn revenue by providing operating reserve services for the transmission system operator.
Some system services may be mutually exclusive depending on the BESS design (e.g., a short duration storage device used to supply regulating reserves would have limited value for deferring transmission or distribution upgrades). Even if a BESS is technically capable of providing multiple services, the additional cycling of the battery (charging and discharging) may degrade the battery and shorten its lifetime and economic viability. Finally, a BESS can only provide a limited duration of any set of services before it runs out of charge, which means batteries must prioritize the services they provide.
Policy examples to increase storage deployment
- Incorporate consideration of storage into grid integration studies to determine the cost-effectiveness of storage relative to other flexibility options at a variety of variable RE penetrations
- Conduct integrated resource planning to identify locations for feasible and effective implementation of storage measures
- Develop modeling tools that can fully characterize the costs and benefits of storage technologies
- Support early-stage research and development into emerging energy storage technologies
- Streamline implementation of new energy storage regulations to reduce administrative delays that limit storage deployment
- Address revenue compensation mechanisms and market shortcomings for the services offered by energy storage resources. These can include:
- Explicitly allowing storage systems to provide system services;
- Ensuring that the unique technical characteristics of storage (fast response time, ability to act as both a load and supply source) are properly compensated;
- Removing barriers to value-stacking.
Reading List
Motivations and Options for Deploying Hybrid Generator-Plus-Battery Projects within the Bulk Power System
Lawrence Berkeley National Laboratory, Electric Power Research Institute, and Clean Kilowatts, 2020
This article explores the advantages, disadvantages, development trends, near-term value proposition, and market participation options for utility-scale hybrid battery projects in the United States, with a focus on PV-battery and wind-battery hybrids. The article is meant to inform electric-sector stakeholders—including industry participants, regulators, market organizers, analysts, and policymakers—who are seeking to understand these types of projects and integrate them into wholesale markets.
Energy Storage in South Asia: Understanding the Role of Grid Connected Energy Storage in South Asia’s Power Sector Transformation
National Renewable Energy Laboratory, 2021
During the last decade, the cost of energy storage technologies has declined rapidly. At the same time, grid flexibility is becoming more important as renewable energy integration increases across the world. Storage technologies have the potential to provide some of this flexibility. However, uncertainties around costs and regulations remain when considering energy storage in India and other South Asia countries, including Bangladesh, Bhutan, and Nepal. This study provides a first-of-its-kind assessment of cost-effective opportunities for grid-scale energy storage deployment in South Asia. The report covers both a near and long term analysis, and discussion of energy storage drivers, potential barriers, and the role of storage in system operations. The state-of-the art modeling approach compares the value of battery storage and pumped hydro storage for 2030 and 2050, considering system operations in India, Bangladesh, Bhutan, and Nepal as a single South Asia interconnection with no institutional barriers to cross-border electricity trade (CBET).
Utility-Scale Battery Storage: Frequently Asked Questions
National Renewable Energy Laboratory, September 2019
This fact sheet provides concise answers to frequently asked questions about utility-scale battery energy storage systems.
The potential for Battery Energy Storage to Provide Peaking Capacity in the United States
National Renewable Energy Laboratory, June 2019
This study examines the potential role of limited-duration battery energy storage in meeting peak demand. As battery storage costs decline, they have become important sources of peak capacity because they reduce net demand. Yet, the economic value of peak capacity storage decreases because peak demand flattens as more storage is added to the system. The paper evaluates the potential market size of peak capacity storage and its sensitivity to various mixes of solar PV and wind. The authors find that this potential strongly depends on the shape of electricity load and grid conditions. Under high penetration of renewable generation, the potential of storage increases substantially. The value of storage doubles when solar PV penetration is greater than 10% whereas the impact of wind is unclear and requires more study.
Battery storage in New Zealand
Transpower New Zealand Limited, September 2017
This study by New Zealand’s grid owner and system operator explores the value of battery energy storage across the electricity supply chain to electricity consumers and the New Zealand electricity system. Findings specific to New Zealand’s electricity market suggest that:
- The greatest value is in behind-the-meter applications
- Existing market tools limit consumer participation in the various energy markets
- The potential value of each service provided by storage varies widely based on physical location and location in the value chain
- Dynamic tariff design such as time-of-use pricing could improve the economic value of batteries
- Behind-the-meter batteries and grid-scale batteries expected to be economic by 2020 and 2022 respectively
The value of energy storage in decarbonizing the electricity sector
Argonne National Laboratory & Massachusetts Institute of Technology, May 2016
This paper examines the value of electric storage in grid decarbonization efforts by using forecasts of hourly electricity demand in Texas in 2035. Electric storage provides a carbon-free source of operational flexibility to the grid by shifting power supplied by variable renewable energy sources, which increases their value to the grid. The authors determine the optimal mix of thermal and renewable resources given various operational limits and assuming different scenarios of installed energy storage capacity and CO2 emission limits. Results suggest that the value of energy storage is only economical under strict emission limits and depends on the availability of flexible nuclear, pumped hydro storage and the share of renewables in the mix.
Energy Storage Requirements for Achieving 50% Solar Photovoltaic Energy Penetration in California
National Renewable Energy Laboratory, 2016
This report estimates the storage required for high PV penetration on the grid (up to 50% in California with renewable penetration over 66%), and quantifies the complex relationships among storage, PV penetration, grid flexibility, and PV costs due to increased curtailment. The authors find that storage needs depend strongly on the amount of other flexibility resources deployed. With very low-cost PV (three cents per kilowatt-hour) and a highly flexible electric power system, about 19 gigawatts of energy storage could enable 50% PV penetration with a marginal net PV levelized cost of energy (LCOE) comparable to the variable costs of future combined-cycle gas generators under carbon constraints.
Market and Policy Barriers to Energy Storage Deployment
Sandia National Laboratory, September 2013
This report details the barriers that restrict the deployment of energy storage technologies in the United States. The findings are based on interviews with stakeholders and review of regulatory filings in four regions roughly representative of the country. The report suggests that while high capital costs remain a barrier to energy storage, deployment is also impacted by regulatory, market (economic), utility and developer business model, cross-cutting, and technology barriers. The report also presents a discussion of possible solutions to address these barriers and a review of initiatives around the country at the federal, regional and state levels. [Also, read an earlier discussion of barriers to storage deployment].
Regulatory and Policy Examples
FERC Order 841: Electric Storage Participation in Markets Operated by Regional Transmission Organizations and Independent System Operators
Federal Energy Regulatory Commission, February 2018
FERC Order 841 is a final rule from the United States Department of Energy’s Federal Energy Regulatory Commission that directs RTOs and ISOs to develop tariffs to integrate electric storage into all electric (capacity, energy, and ancillary service) markets. This rule is expected to usher in the wider use of electric storage and in turn result in higher integration of intermittent and variable onto the grid. The rule applies to all storage capable of both charging from and discharging to the grid, regardless of whether it was a behind-the-meter, distribution, or transmission level system. In May 2019, citing their jurisdictional authority, FERC commissioners declined to allow states to opt out of this rule.
Rulemaking 15-03-011: Decision on Multiple-Use Application Issues
Public Utilities Commission of the State of California, January 2018
Battery storage systems can maximize their value to the grid and to project developers by providing multiple services. This multi-use approach to BESS is known as value stacking. To ensure battery projects can select the most cost-effective combinations of services to provide without negatively impacting the reliability of the grid, California regulators developed 12 rules dictating battery behavior around value-stacking. Among other considerations, these rules ensure batteries:
- Cannot contract for additional services that might interfere with obligations to provide reliability services;
- Meet all performance and availability requirements for the services they provide; and
- Inform the utility of services it currently provides or intends to provide.
Xcel Energy: Guidance No. 1 for the Interconnection of Electric Storage as Stand-Alone Sources, Parallel Operation for Customers without Generation, and in Parallel with Self-Generation
Xcel Energy, January 2017
This document outlines electric storage interconnection guidelines for three different configurations:
- Case 1a: Stand-by energy storage – Provision for facilities that require stand-by (backup) systems to provide power through onsite or grid-charged batteries.
- Case 1b: Parallel Operation without onsite generation – Provision for batteries without onsite generation to operate in parallel with utility
- Case 1c: Parallel Operation with non-net metered, non-renewable onsite generation – Provision for batteries with onsite generation to operate in parallel with utility
To ensure compliance with interconnection rules and prevent unintended export of power to the grid in cases 1b and 1c, meters and the appropriate control systems must be installed and registered.
AB-2514 Energy storage systems
Public Utilities Commission of the State of California, September 2010
California established a target of 1.325 GW of energy storage by 2020 for its various investor-owned utilities. Key details included:
- Specific biennial procurements requirements for each utility. As at June 2018, California’s three main investor-owned utilities - Pacific Gas & Electric, Southern California Edison and San Diego Gas & Electric were 40%, 70% and 95% towards their respective goals of a combined 1.325 GW of battery energy storage.
- Value-stacking of energy storage allowed. That is, energy storage could be used in multiple applications in capacity, ancillary and peak shaving services.
- Utilities’ ownership of storage could not exceed 50%.
- Large scale pumped hydro storage could not be used to meet requirement.